A silicon atom has 14 electrons in total. The electronic configuration of silicon is 1s² 2s² 2p⁶ 3s² 3p². To determine the number of orbitals that are occupied, you can count the unique combinations of quantum numbers for each electron.
In the case of silicon:
- 1s² occupies one s orbital.
- 2s² occupies one s orbital.
- 2p⁶ occupies three p orbitals.
- 3s² occupies one s orbital.
- 3p² occupies three p orbitals.
Adding these up, you get a total of 9 orbitals that are occupied in a silicon atom.
How to Calculate Occupied Orbitals in Silicon Atoms?
Delving into the atomic realm of silicon, a fundamental step involves unraveling the method to calculate its occupied orbitals.
Analyzing the Electron Configuration:
The electron configuration of an atom tells us how the electrons are distributed among its energy levels and sublevels. In the case of silicon, its electron configuration is 1s² 2s² 2p⁶ 3s² 3p².
Counting the Number of Electrons in Each Sublevel:
Let’s break down the electron configuration and count the electrons in each sublevel:
1s²: This sublevel contains 2 electrons.
2s²: This sublevel also contains 2 electrons.
2p⁶: The p sublevel contains 6 electrons.
3s²: The s sublevel contains 2 electrons.
3p²: The p sublevel contains 2 electrons.
Determination of the Occupied Orbitals:
To determine the occupied orbitals, we need to consider the specific sublevels that have electrons:
1s²: The 1s sublevel is fully occupied with 2 electrons.
2s²: The 2s sublevel is fully occupied with 2 electrons.
2p⁶: All three p orbitals (px, py, pz) in the 2p sublevel are occupied with a total of 6 electrons.
3s²: The 3s sublevel is fully occupied with 2 electrons.
3p²: All three p orbitals in the 3p sublevel are occupied with a total of 2 electrons.
Total Number of Occupied Orbitals:
Adding up the occupied orbitals from the above analysis:
1s orbital
2s orbital
2p orbital (px, py, pz)
3s orbital
3p orbital (px, py, pz)
So, we can say the total number of occupied orbitals in a silicon atom is 9.
What is Silicon Atom’s Electron Configuration?
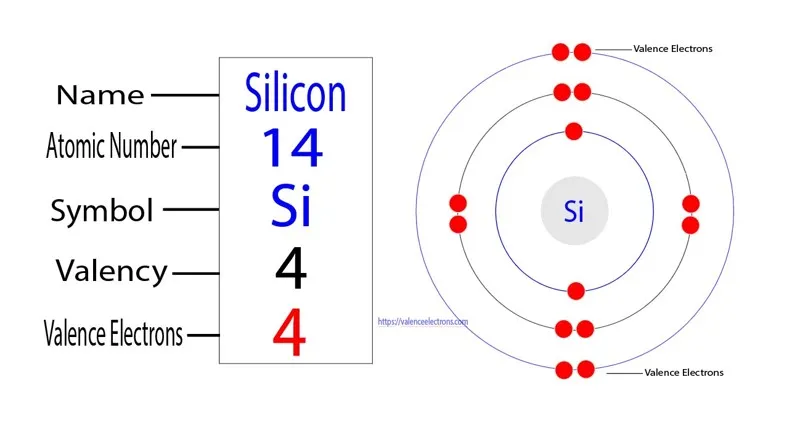
At its core, the electron configuration of a silicon atom provides a roadmap. It is additionally, understanding its occupied orbitals and the arrangement of its electrons within them.
Explanation of Electron Configuration Notation:
Electron configuration notation is a way of representing how electrons are distributed in the various energy levels and sublevels of an atom.
Moreover, it follows a specific format: the principal quantum number (n) indicates the energy level, the letter represents the sublevel (s, p, d, f), and the superscript denotes the number of electrons in that sublevel. For example, “1s²” means there are 2 electrons in the 1s sublevel.
Breakdown of Silicons Electron Configuration:
Silicon’s electron configuration is 1s² 2s² 2p⁶ 3s² 3p². Let’s break it down:
1s²: The first energy level (n=1) has a single sublevel, the 1s sublevel, which can hold up to 2 electrons. This means that the 1s orbital is fully occupied with 2 electrons.
2s² 2p⁶: The second energy level (n=2) has two sublevels: 2s and 2p. The 2s sublevel can hold up to 2 electrons, and the 2p sublevel can hold up to 6 electrons.
In silicon’s case, both the 2s and 2p sublevels are fully occupied, with 2 and 6 electrons respectively.
3s² 3p²: The third energy level (n=3) also has two sublevels: 3s and 3p. Similar to the second energy level, the 3s sublevel can hold up to 2 electrons, and the 3p sublevel can hold up to 6 electrons.
For silicon, both the 3s and 3p sublevels are fully occupied, with 2 and 2 electrons respectively.
Significance of Each Energy Level and Sublevel:
The distribution of electrons into different energy levels and sublevels is crucial because it determines an atom’s chemical and physical properties.
Energy Levels: As the energy level increases (from n=1 to n=3 in silicon’s case), the electrons are progressively farther from the nucleus.
Moreover, electrons in higher energy levels have more energy and are generally involved in chemical reactions.
Sublevels: Sublevels (s, p, d, f) represent distinct shapes and orientations of orbitals within an energy level.
In addition, the s sublevel is spherical, while the p sublevel has three dumbbell-shaped orbitals aligned along different axes. The arrangement of electrons in these sublevels contributes to the atom’s shape and behavior.
How are Electrons Distributed in Orbitals?
Navigating the intricate landscape of electron distribution within orbitals unveils the unique fingerprint of an atom’s chemical behavior.
Orbitals and Sublevels:
Orbitals are regions within an energy level where electrons are most likely to be found. Each energy level is divided into sublevels based on the types of orbitals it contains s, p, d, and f.
s Orbitals: These are spherical orbitals centered around the nucleus. They are the simplest in shape and can hold a maximum of 2 electrons.
p Orbitals: Hence, these are dumbbell-shaped orbitals with three orientations along the x, y, and z axes. Moreover, each p sublevel consists of three p orbitals, each of which can hold 2 electrons, totaling 6 electrons in the p sublevel.
Aufbau Principle and Filling Order of Orbitals:
The Aufbau principle states that electrons fill the lowest energy orbitals first before moving to higher energy ones. This principle guides the order of filling orbitals:
1s: The lowest energy level, 1s, is filled first with 2 electrons.
2s and 2p: The next energy level, 2s, is filled with 2 electrons. Then, the 2p sublevel starts filling, with each orbital initially receiving one electron before any of them get a second electron.
3s and 3p: The third energy level follows a similar pattern, filling the 3s orbital before populating the 3p orbitals.
Pauli Exclusion Principle and Electron Spin:
The Pauli exclusion principle states that no two electrons in an atom can have the same set of quantum numbers. Moreover, this means that each orbital can hold a maximum of 2 electrons, and these electrons must have opposite spins (spin up and spin down).
In addition, electron spin is an intrinsic property that gives each electron a magnetic moment. This principle ensures electron pairing within orbitals.
Hund’s Rule and Electron Pairing Within Orbitals:
Hund’s rule states that electrons will occupy different orbitals within the same sublevel with parallel spins (spin up) before pairing up within the same orbital. .
Moreover, this rule minimizes electron-electron repulsions and stabilizes the atom. For example, in the p sublevel, where there are three orbitals (px, py, and pz), electrons will first singly occupy each orbital with the same spin before pairing up.
What are the Implications and Applications?
The intricacies of occupied orbitals in a silicon atom not only deepens our theoretical understanding of its behavior,
Influence of Silicons Chemical Properties:
The electron configuration of an atom plays a fundamental role in determining its chemical properties. In the case of silicon, its electron configuration (1s² 2s² 2p⁶ 3s² 3p²) has several important implications:
Valence Electrons: The outermost energy level, specifically the 3s² 3p² configuration, defines silicon’s valence electrons. Moreover, these electrons are crucial for forming chemical bonds with other elements.
Tendency to Share Electrons: Silicon’s electron configuration indicates that it has four valence electrons available for bonding.
Additionally, silicon tends to share these electrons with other elements to complete its octet (eight electrons) in the outermost energy level. Moreover, this propensity for covalent bonding is vital in the formation of silicon-based compounds.
Role of Silicon in Semiconductor Technology:
Silicon’s unique electron configuration makes it a critical element in semiconductor technology.
Furthermore, semiconductors are materials that have electrical conductivity between that of conductors (like metals) and insulators (like nonmetals). Here’s how silicon’s electron configuration comes into play:
Tetravalent Nature: Silicon’s four valence electrons enable it to form strong covalent bonds with neighboring silicon atoms.
Moreover, it creates a crystalline lattice structure. Each silicon atom shares one of its valence electrons with a neighboring atom, forming a stable framework.
Energy Gap: Silicon’s electron configuration gives it an energy gap between its valence band and conduction band.
In addition, this energy gap is small enough to allow thermal excitation of electrons to the conduction band but large enough to prevent excessive electron flow.
Moreover, this property is essential for the controlled conduction of electric current in semiconductor devices.
Doping: Silicon’s electron configuration can be modified by introducing impurities, a process known as doping.
By adding small amounts of other elements with different electron configurations, such as phosphorus or boron, the electrical properties of silicon can be intentionally altered, making it either an n-type or p-type semiconductor.
Relationship Between Electron Distribution and Atom’s Behavior:
The way electrons are distributed within an atom’s energy levels and sublevels profoundly affects its behavior:
Chemical Reactivity: The number of valence electrons and their arrangement determine how an atom interacts with other atoms.
Moreover, atoms strive to achieve stable electron configurations, often by sharing or transferring electrons to achieve full energy levels.
Ionization Energy and Electron Affinity: The energy required to remove an electron from an atom (ionization energy) or the energy released when an atom gains an electron (electron affinity) is influenced by electron configuration. Elements with nearly full or nearly empty sublevels tend to have higher ionization energies and lower electron affinities.
Magnetism: Electron spin, a result of the Pauli exclusion principle, contributes to the magnetic properties of materials. Materials with unpaired electrons exhibit magnetic behavior.
FAQ’s
What orbitals are occupied in silicon?
Silicon’s occupied orbitals are 1s², 2s², 2p⁶, 3s², and 3p².
How do you find the number of occupied orbitals?
Count the number of different energy levels and sublevels with electrons in an atom’s electron configuration.
How many subshells are in silicon?
Silicon has 3 subshells: 1s, 2s, and 2p.
Does silicon have d orbitals?
No, silicon does not have occupied d orbitals in its ground state electron configuration.
Why does silicon have d orbitals?
Silicon does not have occupied d orbitals because it falls in the third row of the periodic table and doesn’t reach the d-block elements.
What are all 5 d orbitals?
The five d orbitals are named as dxy, dxz, dyz, dz², and dx²-y². They have distinct shapes and orientations within a subshell.
What are d orbitals?
d orbitals are a type of atomic orbital with complex shapes and orientations, contributing to the electron cloud distribution around the nucleus. They play a role in bonding and electron arrangement in transition metals.
Final Words
In conclusion, understanding the electron configuration of atoms, such as silicon, provides deep insights into their fundamental properties and behaviors.
Moreover, the electron configuration determines how electrons are distributed among energy levels and sublevels, influencing an atom’s chemical reactivity, bonding tendencies, and physical characteristics.
In the case of silicon, its electron configuration (1s² 2s² 2p⁶ 3s² 3p²) shapes its unique role in both chemistry and technology.
The arrangement of electrons in orbitals governs its capacity to form covalent bonds and participate in chemical reactions. Silicon’s significance extends further into the realm of semiconductor technology, where its tetravalent nature, energy gap, and doping capabilities enable the creation of electronic components crucial for modern devices.
In addition, electron configuration is a foundational concept that links the microscopic world of atomic structure to macroscopic material properties.